Mastering ARC in iOS: The Ultimate Interview Guide to Memory Management
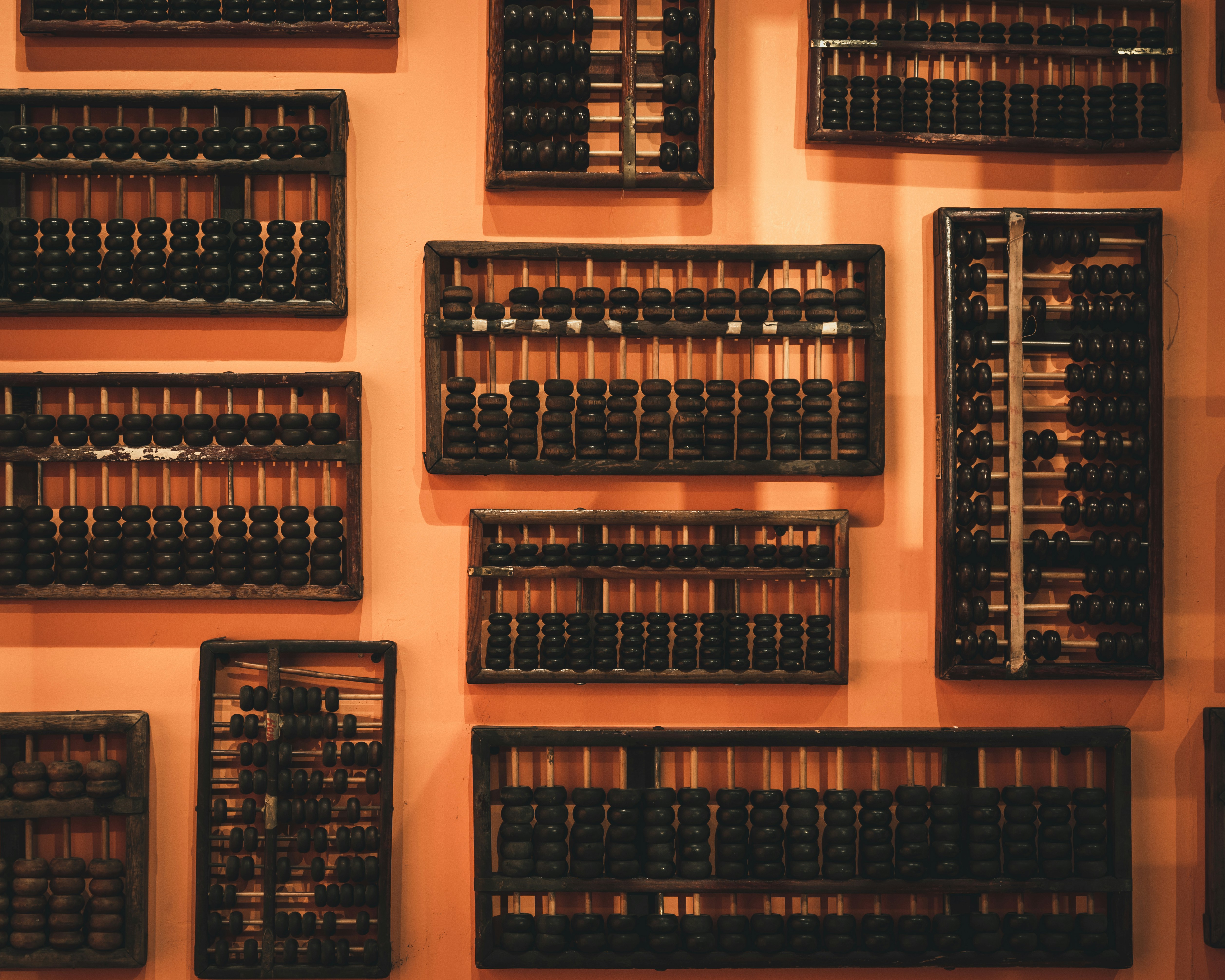
In the ever-evolving world of iOS development, understanding memory management is essential for creating robust and efficient applications. At the heart of this lies Automatic Reference Counting (ARC), a powerful mechanism that simplifies memory handling by automatically inserting retain and release calls. In this article, we dive deep into ARC—from its basic principles and internal mechanics to advanced topics such as autorelease pools, bridging with Core Foundation, and multithreading considerations. Whether you’re prepping for a technical interview or looking to fine-tune your development skills, this guide will equip you with expert insights and practical code examples to master ARC and elevate your iOS development game.
Table of Contents
- What Could You Tell Me About ARC (Automatic Reference Counting)?
- Internal Mechanics of ARC
- Performance Considerations
- Retain Cycles and Their Resolution
- Autorelease Pools and Memory Management
- Bridging with Core Foundation
- Multithreading and Atomicity
- Low-Level Documentation and Source Code
- Summary
1. Whats could you tell me about ARC (Automatic Reference Counting)?
The best ways to answer this question.
Short Answer
Automatic Reference Counting (ARC) is a compile‐time feature used in both Swift and Objective-C that automatically manages an object’s memory by inserting the necessary retain and release calls. This means that when an object is no longer referenced by any strong pointers, ARC deallocates it, reducing the chance of memory leaks without requiring manual intervention.
Detailed Answer
ARC works by maintaining a reference count for every object in your app. When you create an instance of a class, ARC sets its reference count to one. As you assign that instance to other variables, pass it to functions, or store it in collections, ARC increases the count. On the other hand, when a reference is removed (for example, by setting a variable to nil or when the variable goes out of scope), ARC decrements the count. Once the reference count drops to zero, ARC automatically deallocates the object and frees its memory.
A few key points to understand:
Strong References:
These are the default type of reference in Swift. They “own” the object and keep it in memory as long as at least one strong reference exists. (For most relationships, strong references are sufficient.)
Weak and Unowned References:
To avoid retain cycles (situations where two objects hold strong references to each other, preventing deallocation) you can declare some references as weak or unowned.
Weak references do not increase the object’s reference count and automatically become nil when the object is deallocated.
In many cases, such as implementing a delegate pattern, you want to avoid retain cycles between two objects. In this example, the DownloadManager class holds a weak reference to its delegate, which is implemented by a view controller. This ensures that if the view controller is deallocated, the delegate reference is automatically set to nil.
1
2
3
4
5
6
7
8
9
10
11
12
13
14
15
16
17
18
19
20
21
22
23
24
25
26
27
28
29
30
31
32
33
34
35
36
37
38
39
protocol DownloadManagerDelegate: AnyObject {
func downloadDidFinish(_ manager: DownloadManager)
}
class DownloadManager {
// The delegate is weak to avoid a retain cycle.
weak var delegate: DownloadManagerDelegate?
func startDownload() {
print("Download started...")
// Simulate a download delay
DispatchQueue.global().asyncAfter(deadline: .now() + 2) { [weak self] in
guard let self = self else { return }
// Notify the delegate on the main thread
DispatchQueue.main.async {
self.delegate?.downloadDidFinish(self)
}
}
}
}
class ViewController: DownloadManagerDelegate {
let downloadManager = DownloadManager()
init() {
// Assign self as the delegate (using weak reference avoids a retain cycle)
downloadManager.delegate = self
downloadManager.startDownload()
}
func downloadDidFinish(_ manager: DownloadManager) {
print("Download finished! Updating UI accordingly.")
}
}
// Usage
var viewController: ViewController? = ViewController()
// Later, if the view controller is dismissed:
viewController = nil
Unowned references also don’t increase the count, but they assume the referenced object will always be valid (accessing them after deallocation leads to a runtime error).
When you have two objects where one (e.g., a credit card) always has a valid owner (e.g., a customer), you can use an unowned reference. In this example, a CreditCard instance holds an unowned reference to its Customer. Since a credit card should not outlive its customer, it’s safe to use an unowned reference.
1
2
3
4
5
6
7
8
9
10
11
12
13
14
15
16
17
18
19
20
21
22
23
24
25
26
27
28
29
30
31
32
33
34
35
36
class Customer {
let name: String
var card: CreditCard?
init(name: String) {
self.name = name
print("\(name) is being initialized")
}
deinit {
print("\(name) is being deinitialized")
}
}
class CreditCard {
let number: String
// Unowned reference assumes the customer will always exist while the card exists.
unowned let customer: Customer
init(number: String, customer: Customer) {
self.number = number
self.customer = customer
print("CreditCard \(number) is being initialized")
}
deinit {
print("CreditCard \(number) is being deinitialized")
}
}
// Usage
var customer: Customer? = Customer(name: "Alice")
customer?.card = CreditCard(number: "1234-5678-9012-3456", customer: customer!)
customer = nil
// Both Customer and CreditCard are deallocated, avoiding any retain cycle.

Compiler Optimizations:
Unlike garbage collection, which periodically scans for unused objects at runtime, ARC’s operations are inserted at compile time. This results in predictable performance and lower overhead. However, ARC does not automatically resolve retain cycles. You, as the developer, must break those cycles (often using weak or unowned references).
In summary, ARC simplifies memory management by automating the retain/release process, ensuring efficient deallocation of objects when they’re no longer needed. This allows developers to focus more on business logic while still maintaining robust control over memory usage. Understanding ARC, its reference types, and potential pitfalls like retain cycles is fundamental for writing high-quality iOS applications. 
2. Internal Mechanics of ARC
ARC’s internal mechanics go far beyond the simple idea of “automatic” retain and release calls; they involve a sophisticated compile‐time process that inserts memory management calls into your code based on a detailed static analysis. Here’s an explanation along with some examples:
Static Analysis & Code Insertion:
During compilation, the Swift compiler analyzes your code to determine where object references are created and destroyed. It automatically inserts calls equivalent to swift_retain() and swift_release() at points where variables are assigned, passed as arguments, or go out of scope.
Two-Pass Process:
Conceptually, ARC works in two phases. In the first phase, it inserts all necessary retain and release calls to preserve program semantics. In the second phase, it optimizes away redundant calls through flow analysis (for example, if an object is created and immediately discarded, the compiler may eliminate unnecessary retain/release pairs).
Reference Counting & Life Cycle:
Every instance of a class is allocated on the heap and associated with a reference count. When you assign an instance to a variable, ARC increases the reference count (retain); when that variable goes out of scope or is set to nil, ARC decreases the count (release). When the count reaches zero, the object is deinitialized and its memory is reclaimed.
This example demonstrates how ARC automatically manages the memory of a simple class by inserting retain and release calls behind the scenes. Although you don’t see the low-level calls, the output reflects the life cycle:
1
2
3
4
5
6
7
8
9
10
11
12
13
14
15
16
17
18
19
20
21
22
23
class Person {
let name: String
init(name: String) {
self.name = name
print("\(name) was initialized")
}
deinit {
print("\(name) was deallocated")
}
}
func runScenario() {
let person = Person(name: "John")
// 'person' is retained within this scope.
// When this function ends, the reference count drops to 0 and the object is deallocated.
}
runScenario()
// Expected output:
// John was initialized
// John was deallocated
Weak References and Side Tables:
For weak references, ARC uses an additional mechanism called “side tables.” Since most objects don’t have weak references, it’s inefficient to store extra counters in every object. Instead, when a weak reference is created, ARC allocates a side table to track the weak reference count. When the object is deallocated, ARC “zeroes out” any weak references (i.e., sets them to nil) by consulting the side table.
This example shows how weak references work. The weak reference does not increase the reference count and is automatically set to nil when the object is deallocated:
1
2
3
4
5
6
7
8
9
10
11
12
13
14
15
16
17
18
19
20
21
22
23
24
25
26
27
28
29
30
31
32
33
34
35
36
37
38
39
40
41
42
class Teacher {
let name: String
init(name: String) {
self.name = name
print("Teacher \(name) was initialized")
}
deinit {
print("Teacher \(name) was deallocated")
}
}
class Student {
let name: String
// Weak reference to avoid a strong reference cycle.
weak var teacher: Teacher?
init(name: String) {
self.name = name
print("Student \(name) was initialized")
}
deinit {
print("Student \(name) was deallocated")
}
}
var teacher: Teacher? = Teacher(name: "Mr. Smith")
var student: Student? = Student(name: "Alice")
student?.teacher = teacher
// When we set teacher and student to nil, both objects are deallocated.
teacher = nil
student = nil
// Expected output:
// Teacher Mr. Smith was initialized
// Student Alice was initialized
// Teacher Mr. Smith was deallocated
// Student Alice was deallocated
Unowned References:
Unowned references, similar to weak ones, do not increase the reference count; however, they assume that the referenced object will always be valid during their lifetime. If you access an unowned reference after the object has been deallocated, a runtime error occurs. The compiler treats these differently, bypassing the side table overhead but requiring you to be certain about the lifetime relationships.
When one object is guaranteed to outlive another, you can use an unowned reference. In this example, a CreditCard always has an associated Customer. The CreditCard holds an unowned reference to its Customer.
1
2
3
4
5
6
7
8
9
10
11
12
13
14
15
16
17
18
19
20
21
22
23
24
25
26
27
28
29
30
31
32
33
34
35
36
37
38
39
40
class Customer {
let name: String
var card: CreditCard?
init(name: String) {
self.name = name
print("Customer \(name) was initialized")
}
deinit {
print("Customer \(name) was deallocated")
}
}
class CreditCard {
let number: String
// Unowned reference assumes the customer will always be valid while the card exists.
unowned let customer: Customer
init(number: String, customer: Customer) {
self.number = number
self.customer = customer
print("CreditCard \(number) was initialized")
}
deinit {
print("CreditCard \(number) was deallocated")
}
}
// Usage
var customer: Customer? = Customer(name: "Alice")
customer?.card = CreditCard(number: "1234-5678-9012-3456", customer: customer!)
customer = nil
// Expected output:
// Customer Alice was initialized
// CreditCard 1234-5678-9012-3456 was initialized
// CreditCard 1234-5678-9012-3456 was deallocated
// Customer Alice was deallocated
Additional Insights on ARC’s Internal Mechanics
Optimization:
ARC’s static analysis not only inserts memory management calls but also optimizes them. For example, if an object’s value is never used after creation, ARC might eliminate unnecessary retain/release pairs. This optimization minimizes runtime overhead.
Compiler Integration:
The Swift compiler’s SIL (Swift Intermediate Language) generation phase is where ARC decisions are made. The compiler translates your high-level code into SIL, inserting calls like swift_retain() and swift_release() based on its analysis.
Side Tables:
Side tables are used only when weak references are present. They store extra metadata (like the weak reference count) without increasing the size of every object, thus conserving memory.
Unowned vs. Weak:
While both unowned and weak references don’t affect the strong reference count, unowned references are non-optional and don’t use a side table. They’re used when you are absolutely sure that the referenced object will outlive the reference, offering a slight performance benefit over weak references.
3. Performance Considerations:
When it comes to performance, not all reference types in ARC are created equal.
Weak References:
Weak references are implemented using side tables. Every time you access a weak reference, ARC must perform an extra lookup in this side table to check if the referenced object is still alive (i.e., not deallocated). Although this overhead is generally very small, in performance-critical code with a very high frequency of weak reference accesses (such as inside a tight loop), it may add measurable overhead.
In this example, the ManagerWeak class holds a weak reference to a Worker. Each time the worker is accessed in the loop, ARC performs a side table lookup to ensure that the reference is still valid. While this check is fast, it still adds a slight overhead when performed millions of times.
1
2
3
4
5
6
7
8
9
10
11
12
13
14
15
16
17
18
19
20
21
22
class Worker {
func performTask() {
// Simulate a task
}
}
class ManagerWeak {
// 'worker' is a weak reference, so accessing it involves a side table lookup.
weak var worker: Worker?
func execute() {
for _ in 0..<1_000_000 {
worker?.performTask()
}
}
}
// Usage
let workerInstance = Worker()
var managerWeak = ManagerWeak()
managerWeak.worker = workerInstance
managerWeak.execute()
Unowned References:
Unowned references, in contrast, do not use side tables. They are stored directly as a pointer to the object, so accessing an unowned reference is faster since there’s no extra indirection. However, they come with the risk that if the referenced object is deallocated and you attempt to access the unowned reference, your program will crash. Therefore, unowned references should only be used when you can guarantee that the referenced object will outlive the reference.
In this case, each call to worker.performTask() directly accesses the memory pointer without an extra lookup. This makes the unowned reference slightly more performant, especially in scenarios with intensive reference accesses. However, you must ensure that the Worker instance remains alive for as long as ManagerUnowned needs it.
1
2
3
4
5
6
7
8
9
10
11
12
13
14
15
16
17
18
19
class ManagerUnowned {
// 'worker' is an unowned reference, so it is accessed directly.
unowned var worker: Worker
init(worker: Worker) {
self.worker = worker
}
func execute() {
for _ in 0..<1_000_000 {
worker.performTask()
}
}
}
// Usage
let workerInstance2 = Worker()
var managerUnowned = ManagerUnowned(worker: workerInstance2)
managerUnowned.execute()
Summary
- Weak references are safer because they automatically become nil when the referenced object is deallocated, but they incur a small performance cost due to the side table lookup.
- Unowned references are more performant because they are direct pointers, but they require you to guarantee that the referenced object will remain valid during the unowned reference’s lifetime. Failure to do so can lead to runtime crashes.
4. Retain Cycles and Their Resolution:
Retain cycles occur when two or more objects hold strong references to each other, preventing ARC from deallocating them—even if no other parts of your code need these objects—leading to memory leaks. This problem is especially common in complex object graphs and with closures that capture self. Here’s a closer look at common scenarios, techniques to resolve them, and how to detect these issues
Common Retain Cycle Scenarios
Two Objects Referencing Each Other:
When two instances hold strong references to each other, neither can be deallocated. For example, consider a Person and an Apartment where each keeps a reference to the other.
Problematic Code (Retain Cycle):
1
2
3
4
5
6
7
8
9
10
11
12
13
14
15
16
17
18
19
20
21
22
23
24
25
26
27
28
29
30
31
32
33
34
35
36
37
38
class Person {
let name: String
var apartment: Apartment? // Strong reference
init(name: String) {
self.name = name
print("\(name) was initialized")
}
deinit {
print("\(name) was deallocated")
}
}
class Apartment {
let unit: String
var tenant: Person? // Strong reference
init(unit: String) {
self.unit = unit
print("Apartment \(unit) was initialized")
}
deinit {
print("Apartment \(unit) was deallocated")
}
}
var person: Person? = Person(name: "John")
var apartment: Apartment? = Apartment(unit: "4A")
person!.apartment = apartment
apartment!.tenant = person
// Even if we set person and apartment to nil,
// they won’t be deallocated because they hold strong references to each other.
person = nil
apartment = nil
Resolution:
To break the cycle, one of the references should be declared as weak (or unowned if appropriate). Typically, you’ll make the back reference weak:
1
2
3
4
5
6
7
8
9
10
11
12
13
14
class Apartment {
let unit: String
// Use a weak reference to avoid a retain cycle
weak var tenant: Person?
init(unit: String) {
self.unit = unit
print("Apartment \(unit) was initialized")
}
deinit {
print("Apartment \(unit) was deallocated")
}
}
Retain Cycles in Closures:
Closures capture variables (including self) by default as strong references. If you assign a closure to a property of a class instance and it captures that instance, you create a cycle where the instance holds the closure and the closure holds the instance.
Problematic Code:
1
2
3
4
5
6
7
8
9
10
11
12
13
14
15
16
17
18
19
20
class HTMLElement {
let name: String
// The closure captures self strongly by default
lazy var asHTML: () -> String = {
return "<\(self.name)>Hello, world!</\(self.name)>"
}
init(name: String) {
self.name = name
print("\(name) was initialized")
}
deinit {
print("\(name) was deallocated")
}
}
var element: HTMLElement? = HTMLElement(name: "p")
print(element!.asHTML())
element = nil // The HTMLElement will never be deallocated because the closure retains self.
Resolution with Capture List:
Use a capture list to break the cycle. Often you’ll capture self weakly (or unowned if you’re sure it will always be valid).
1
2
3
4
5
6
7
8
9
10
11
12
13
14
15
16
17
18
19
20
class HTMLElement {
let name: String
lazy var asHTML: () -> String = { [weak self] in
guard let self = self else { return "" }
return "<\(self.name)>Hello, world!</\(self.name)>"
}
init(name: String) {
self.name = name
print("\(name) was initialized")
}
deinit {
print("\(name) was deallocated")
}
}
var element: HTMLElement? = HTMLElement(name: "p")
print(element!.asHTML())
element = nil // Now, the HTMLElement instance is deallocated.
Detecting and Resolving Retain Cycles
- Xcode’s Memory Graph Debugger: Use the Memory Graph Debugger (accessed via Xcode’s Debug navigator) to visualize object relationships. It shows you which objects are still in memory and how they reference each other. Retain cycles will appear as groups of objects that reference each other, preventing deallocation.
- Instruments – Leaks Tool: Instruments’ Leaks tool can help detect memory leaks. Running your app with Instruments can reveal objects that are never deallocated, often pointing to a retain cycle.
- Code Reviews & Static Analysis: Regular code reviews and using Xcode’s static analyzer can also help catch potential retain cycles before they become issues in production.
5. Autorelease Pools and Memory Management:
Under ARC, most objects are deallocated as soon as their strong references go out of scope. However, when working with certain Objective-C APIs or creating a lot of temporary objects (for example, inside a tight loop or on a background thread), some objects are placed into an autorelease pool. An autorelease pool collects these temporary objects and defers their release until the pool is drained, which typically happens at the end of the current run loop iteration. This can lead to higher memory usage if not managed properly.
Why Use Explicit Autorelease Pools?
Memory-Intensive Loops: In a loop that creates many temporary objects (especially when using APIs that return autoreleased objects), those objects are held in the default autorelease pool until the end of the run loop. By wrapping the loop body in an explicit autorelease pool, you force the pool to drain each iteration, releasing memory sooner.
Imagine you’re processing a large number of images. Each iteration might create temporary objects (e.g., images loaded from disk) that are autoreleased. Without an explicit pool, these objects would accumulate until the end of the run loop.
1
2
3
4
5
6
7
8
9
10
11
for i in 0..<1000 {
autoreleasepool {
// Suppose loadImage returns an autoreleased UIImage (for example, via an Objective-C API)
if let image = UIImage(named: "Image_\(i)") {
// Process the image (e.g., resizing or filtering)
print("Processing image \(i)")
}
}
// The autorelease pool drains at the end of each iteration,
// releasing the temporary objects created within the block.
}
- Background Threads: Background threads do not have an automatically created autorelease pool. If you’re running code on a background thread that creates autoreleased objects, you should wrap that code in an autorelease pool to ensure timely deallocation.
When performing tasks on a background thread—especially if you’re interfacing with Objective-C APIs—you may need to create an explicit autorelease pool since one isn’t provided by default.
1
2
3
4
5
6
7
8
9
10
11
DispatchQueue.global(qos: .background).async {
autoreleasepool {
// Memory-intensive work on a background thread
for i in 0..<500 {
// Create an autoreleased temporary object
let tempString = NSString(format: "Processing item %d", i)
print(tempString)
}
}
// Once the autorelease pool block is exited, all temporary objects are released.
}
6. Bridging with Core Foundation:
Bridging between ARC-managed objects (like those in Objective-C or Swift) and Core Foundation objects (such as CFString, CFArray, etc.) lets you work with both memory management models safely. Since Core Foundation uses manual reference counting while ARC handles memory automatically, you must tell the compiler when ownership is being transferred between the two systems. This is where the __bridge, __bridge_transfer, and __bridge_retained casts come into play.
Key Concepts
__bridge: Use __bridge when you want to cast an object between Core Foundation and Objective-C (or Swift) without transferring ownership. The object’s memory management remains unchanged. You are simply telling the compiler to treat the object as a different type.
Suppose you create a Core Foundation string and want to use it as an NSString without changing its ownership. Here, __bridge tells the compiler to simply reinterpret cfString as an NSString. The object is still owned by Core Foundation, so you must call CFRelease when done.
1
2
3
4
5
6
7
8
9
// Create a CFStringRef (Core Foundation object)
CFStringRef cfString = CFStringCreateWithCString(kCFAllocatorDefault, "Hello, world!", kCFStringEncodingUTF8);
// Cast to NSString without transferring ownership
NSString *nsString = (__bridge NSString *)cfString;
NSLog(@"%@", nsString);
// Since ownership wasn't transferred, you must manually release the CF object.
CFRelease(cfString);
__bridge_transfer: Use __bridge_transfer when you want to transfer ownership of a Core Foundation object to ARC. ARC will then take over responsibility for releasing the object automatically. This is commonly used when a function creates a CF object (for example, using a “Create” or “Copy” function) and you want to convert it into an ARC-managed object.
Now, if you want ARC to manage the lifetime of the CF object, use __bridge_transfer. With __bridge_transfer, the ownership of cfString is transferred to ARC. ARC now automatically releases nsString when it goes out of scope.
1
2
3
4
5
6
7
8
// Create a CFStringRef that you want to hand over to ARC
CFStringRef cfString = CFStringCreateWithCString(kCFAllocatorDefault, "Hello, ARC!", kCFStringEncodingUTF8);
// Transfer ownership to ARC: ARC will now manage and eventually release this object.
NSString *nsString = (__bridge_transfer NSString *)cfString;
NSLog(@"%@", nsString);
// No need to call CFRelease here; ARC will take care of it.
__bridge_retained: Use __bridge_retained when transferring an ARC-managed object to a Core Foundation context. This tells the compiler to “retain” the object in the Core Foundation world, meaning you become responsible for releasing it manually (with CFRelease) later on.
Sometimes you need to pass an ARC-managed object to a Core Foundation API. In this case, use __bridge_retained. Here, __bridge_retained moves the object from ARC management to manual management in the Core Foundation world. You must call CFRelease to avoid memory leaks.
1
2
3
4
5
6
7
8
9
10
11
12
// Create an ARC-managed NSString
NSString *nsString = [[NSString alloc] initWithCString:"Hello, CF!" encoding:NSUTF8StringEncoding];
// Transfer ownership from ARC to Core Foundation.
// The returned CFStringRef now has a +1 retain count and must be released manually.
CFStringRef cfString = (__bridge_retained CFStringRef)nsString;
// Use cfString with a Core Foundation API...
CFShow(cfString);
// Since ownership was transferred, release the CF object manually.
CFRelease(cfString);
7. Multithreading and Atomicity:
ARC’s reference counting operations are thread-safe—meaning that the increments and decrements to an object’s reference count are performed atomically even when accessed from multiple threads. However, this thread-safety only applies to memory management itself; it does not guarantee that the objects you’re managing are safe to access or modify concurrently. When multiple threads modify a shared object without proper synchronization, race conditions can occur.
Key Points
- ARC Thread-Safety: ARC ensures that retain and release operations are atomic. This prevents memory management crashes (such as over-releasing an object) when objects are accessed across threads.
- Concurrent Access Issues: Although ARC’s reference counting is thread-safe, the object’s internal state isn’t automatically protected. If multiple threads read from or write to an object concurrently, you may encounter race conditions, data corruption, or crashes.
- Atomicity vs. Thread-Safety: ARC makes the retain/release calls atomic, but it doesn’t make the object’s properties or methods thread-safe. To safely modify shared data, you must use additional synchronization mechanisms (e.g., serial dispatch queues, locks, or other thread-safety techniques).
Concurrent Access Without Synchronization
Consider a simple counter class that is accessed concurrently from multiple threads without proper synchronization. Even though ARC handles memory management safely, concurrent writes to the counter can lead to a race condition. As a result, the final value may be less than 1,000 due to race conditions.
1
2
3
4
5
6
7
8
9
10
11
12
13
14
15
16
class Counter {
var value: Int = 0
func increment() {
value += 1
}
}
let counter = Counter()
// Simulate concurrent increments on a global concurrent queue
DispatchQueue.concurrentPerform(iterations: 1000) { _ in
counter.increment()
}
print("Counter value: \(counter.value)")
Protecting Shared Data with a Serial Dispatch Queue
To avoid race conditions, you can synchronize access to the shared object. One common approach is to use a serial dispatch queue to ensure that only one thread accesses or modifies the shared resource at a time. Both read and write operations are executed on this queue, ensuring that increments occur in a controlled manner without interference from other threads. This guarantees that the final counter value is correct.
1
2
3
4
5
6
7
8
9
10
11
12
13
14
15
16
17
18
19
20
21
22
23
24
class SafeCounter {
private var _value: Int = 0
private let queue = DispatchQueue(label: "com.example.SafeCounter")
var value: Int {
// Synchronize read access on the serial queue.
return queue.sync { _value }
}
func increment() {
// Synchronize write access on the serial queue.
queue.sync {
_value += 1
}
}
}
let safeCounter = SafeCounter()
DispatchQueue.concurrentPerform(iterations: 1000) { _ in
safeCounter.increment()
}
print("SafeCounter value: \(safeCounter.value)")
Even with ARC’s safety for memory management, neglecting synchronization for shared mutable data can lead to race conditions and data corruption. Always analyze your multithreaded code to ensure that concurrent modifications are properly synchronized.
By understanding both ARC’s thread-safety guarantees and the limitations regarding object-level concurrency, you can write more robust and safe multithreaded code in your iOS applications.
8. Low-Level Documentation and Source Code:
For the truly curious, digging into low‑level documentation and source code can reveal the inner workings of ARC beyond its everyday usage. Here are some official resources to help you dive deeper into the low-level aspects of ARC and memory management in Swift.
- Swift Programming Language – Automatic Reference Counting
Learn how ARC works in Swift, including its fundamentals and how the compiler manages memory automatically.
https://docs.swift.org/swift-book/documentation/the-swift-programming-language/automaticreferencecounting/ - Swift Open Source Repository
Explore the Swift source code on GitHub, including the implementation of reference counting and other runtime details.
https://github.com/apple/swift - Clang Documentation on Automatic Reference Counting
Although focused on Objective‑C, this documentation explains how ARC was designed and implemented at the compiler level, which is closely related to Swift’s approach.
https://clang.llvm.org/docs/AutomaticReferenceCounting.html - Apple Developer Memory Management Guides
While some of these guides are older, they provide a solid background on memory management principles in Cocoa and can offer insights into ARC’s evolution.
https://developer.apple.com/library/archive/documentation/Cocoa/Conceptual/MemoryMgmt/Articles/mmPractical.html
Summary
We’ve explored the multifaceted nature of ARC, unraveling how it automatically manages memory through reference counting and the insertion of retain/release calls during compilation. We examined the roles of strong, weak, and unowned references, and how to resolve common pitfalls like retain cycles—especially in complex object graphs and closures. Additionally, we delved into the nuances of autorelease pools, bridging between ARC and Core Foundation, and the implications of multithreading on memory safety. By mastering these concepts and utilizing the provided resources, you’re well on your way to not only acing your technical interviews but also building more reliable and efficient iOS applications.